I. Introduction
Many nations are striving to secure military superiority in space (Kim, 2020). While it is unclear how they are managing the safety of space force strength, the emergence of various space-related civilian companies that are diligently addressing safety issues in the space industry, with significant focus on establishing international safety standards and developing emergency procedures (IAASS, 2010).
The Republic of Korea’s Ministry of National Defense defines safety management as the effort to protect the lives and physical well-being of defense personnel, as well as national defense assets, from various accidents. It classifies a total of six types of accidents as defense safety accidents, including space-related accidents (MND, 2020).
The current concept of operating space force capabilities is based on utilizing satellites to conduct and command ground, maritime, aerial, and cyber warfare strategies and tactics. As a result, it does not involve operators boarding spacecraft or conducting operations directly in space but rather achieving military objectives through the remote control and management of satellites. Consequently, the primary focus of space force safety management is expected to include preventing collisions between friendly satellites and orbital debris, as well as addressing operational errors by space force agents (Moura & Blamont, 2015).
Historically, approximately 70% of aviation accidents have been attributed to human factors involving operators (Byun, 2004). Safety management strategies for addressing operational errors by space force members can also be explored by drawing lessons from aviation safety. The International Civil Aviation Organization (ICAO) has instructed its member states to implement Safety Management Systems (SMS) through annexes to the International Civil Aviation Convention (ICAO, 2013). The Swiss Cheese Model, applicable to large organizations such as the military for accident prevention, illustrates the paradigm of Organizational Accidents. It explains that accidents occur when “Latent Conditions,” which exist in a dormant state until certain operational situations arise, intersect with Human Failures, referred to as “Active Failures.” (Reason, 1997). The purpose of this study is to suggest safety management measures for national defense space operations based on lessons leaned from Swiss Cheese Model.
II. Review of Previous Studies
Space is currently at the forefront of statecraft. Major powers are channeling their national resources to secure space force dominance. However, there is a lack of clarity regarding how nations are ensuring the safety of their space military capabilities.
Plans for the military utilization of space are rapidly increasing the number of satellites in orbit, far exceeding the approximately 2,000 currently operational satellites, thereby exacerbating disorder and increasing risks. In this situation, nations are adopting mutually competitive stances focused solely on securing their own respective military capabilities, rather than prioritizing efforts to ensure safety in the evolving space environment (Kim, 2019).
Meanwhile, the private sector has seen the emergence of various space-related commercial enterprises such as SpaceX, Blue Origin, and OneWeb, which are rapidly advancing into space, pushing past technological boundaries. These space-related companies are deeply concerned with safety issues that impact the space industry, emphasizing the importance of establishing international safety standards and developing emergency procedures. In the civil section, space safety encompasses protecting the general public from space objects, safeguarding personnel at launch sites, ensuring the safety of spacecraft crew members, ground-based operational facilities, and preserving orbital assets such as satellites and spacecraft. Key initiatives in the civilian space industries aimed at ensuring the safety of commercial space activities on a global level include the followings (IAASS, 2010).
Firstly, protecting civilians from potential risks associated with the launch, flight, and re-entry of space objects.
Secondly, promoting the development, establishment, and operation of safe space systems based on ground and aviation safety regulations, as well as the accumulated experience and knowledge of countries participating in space exploration.
Thirdly, establishing international control regulations to prevent collisions during the launch, orbital flight, or re-entry of space objects with other space systems or aircraft.
Fourthly, fostering collaboration to protect the space and Earth environments from chemical, radiation, and debris-related impacts that may arise during space operations.
Fifthly, preventing intentional destruction of orbital space systems or harmful activities.
Sixthly, ensuring the capability to respond effectively to emergency situations that may arise during space missions.
However, the risk standards for space safety are not well known, primarily because only a few specific countries consider the risk acceptance criteria and mitigation measures during the launch and re-entry of space vehicles. Additionally, incidents where safety requirements were not met but did not result in accidents are often kept confidential. Furthermore, safety-related risk assessments are typically conducted on a case-by-case basis for individual launches or re-entries, without considering correlations with previous launch or re-entry cases, which further limit the understanding of comprehensive risk standards (Choi, 2022).
Uncontrolled or out-of-orbit space debris is generally excluded from risk assessment models, but if such debris falls to Earth, it has the potential to cause substantial damage. In 2014, more than 30,000 pieces of space debris were being tracked, but millions of smaller fragments remain in orbit, beyond the scope of current monitoring capabilities. These debris include dead satellites, rocket components, metal fragments, exploded satellite remains, optical lens covers, and paint chips. These objects can remain in Earth’s orbit for centuries or even millennia, posing a serious risk of significant damage due to their high relative speeds in the event of a collision with other space objects.
NASA (2017) said that they has established and currently implements Space Safety Standards and Procedures, providing detailed regulations and protocols for the safe operation of space programs through various types of NPDs (NASA Policy Directives) and NPRs (NASA Procedural Requirements).
The Republic of Korea’s Ministry of National Defense defines “Safety” in relation to national defense as a condition in which the risk of causing human casualties or property damage is maintained below an acceptable level through the continuous identification of hazards and the implementation of an effective risk management. A “Safety accident” is defined as an event in which unintended causes lead to harm of defense personnel or assets, or cause damage to public or civilian entities during the performance of defense missions or unit activities. “Safety management” is defined as activities aimed at ensuring the safety of defense personnel’s lives and physical well-being, as well as the protection of defense assets from disasters or various other safety incidents
Additionally, the Ministry of National Defense has established definitions and detailed classification criteria for various types of safety accidents. The six categories of defense safety accidents include aviation accidents, weapon and hazardous material accidents, vehicle accidents, ground accidents, water and maritime accidents, and space accidents. The specific subcategories for each type of accident are outlined in Table 1 (MND, 2020).
In the case of the United States, the Department of Defense defines a safety accident as an unintentional or sequential situation that results in damage to Department of Defense (DoD) assets, occupational illnesses among DoD military personnel, on-duty or off-duty physical injuries to DoD military personnel, work-related injuries to DoD civilian employees, damage to public or civilian property caused by DoD activities, or physical injuries or illnesses affecting individuals who are not DoD personnel (DoD, 2011). The U.S. Army defines a safety accident as an unintentional event that leads to death, injury, or illness of individuals, or causes damage to or loss of equipment or property (U.S. Army, 2015). The U.S. Air Force defines a safety accident as an unintentional or sequential event that results in property damage or personal injury classified as Class A, B, C, D, or E. It categorizes and manages safety accidents into seven types: Space, Aviation, Weapons, Afloat, Motor vehicle, Ground, and Miscellaneous (U.S. Air Force, 2020).
Meanwhile, statutory law in Korea also define safety accidents based on their legislative purposes. The Framework Act on Disaster and Safety Management defines “Disasters (a large-scale safety accident)” as events that cause or have the potential to cause harm to the lives, bodies, and property of citizens, or damage to the nation (MOIS, 2024). The Act on Special Cases Concerning the Settlement of Traffic Accidents defines a “traffic accident” as an incident involving a vehicle that results in injury or death to a person or damage to the property (KNPA, 2017). The Aviation Safety Act defines an “Aviation accident” as an occurrence related to the operation of an aircraft, which can happen from the time a person boards on the aircraft with the intention of flight until all passengers have disembarked. It includes cases involving death, serious injury, or disappearance of individuals; damage or structural failure of the aircraft; or situations where the aircraft’s location cannot be determined or it is inaccessible (MOLIT, 2024).
According to the previous reviews, a safety accident can be characterized as an event causing human or material damage in unintended circumstances. National defense safety management, which encompasses space accidents, involves identifying risk factors and applying mitigation measures to safeguard human and material assets during national defense operations.
Space is a domain where numerous countries launch and test satellites, space shuttles, and other spacecraft (Lee, 2021). Space activities encompass a wide range of operations, including launching satellites into orbit, space exploration, human transportation to space, and re-entry to Earth. Consequently, space safety management in the civil section also addresses diverse elements such as the mechanical and physical stability of launch vehicles, safety of launch sites and ground operation facilities, management of spacecraft crew, and control of space launch vehicles (IAASS, 2010).
However, space activities in the military field can be viewed as the operation of space power, involving activities such as information gathering, surveillance and reconnaissance, movement tracking, communications, drone operation, GPS-guided weapons, and support for cyber warfare, all of which rely on satellite utilization (Kim, 2020). In other words, these activities can be described as strategic and tactical military activities utilizing satellites.
Space safety refers to the protection of critical or high-value space systems and infrastructure, making it a fundamental requirement for sustainable space developments and operations. It is also defined as the ability to respond to unintentional threats, including design errors, natural hazards, and human errors (Yim, 2022). Design faults or natural hazards encompass threats such as collisions with orbital debris, commonly known as space junk (Jung, 2015). Human error, meanwhile, may occur during the operation, command, and control of ground, maritime, aerial, and cyber warfare systems that rely on satellite technology.
Furthermore, based on the definition outlined in South Korea’s National Defense Safety Directive, the safety management refers to activities aimed at protecting defense personnel and assets from safety accidents caused by unintentional causes. Thus, intentional actions by adversarial forces, such as attacking military satellites through physical impact or cyber hacking, should be considered outside the scope of safety management and classified separately. Therefore, the scope of safety management for the Republic of Korea’s space force strength would include preventing collisions between military satellites and orbital debris, as well as addressing operational errors caused by human factors such as an operators’ lack of situation awareness or insufficient knowledge of practice.
Historically, approximately 70% of accidents in the aviation industry have been caused by factors related to human error associated with operators (Kim et al., 2015). Therefore, the safety management of space force strength can also draw on lessons from aviation safety to explore effective strategies.
The International Civil Aviation Organization (ICAO) defines “Safety” as the state in which risks that could harm people or property are managed and reduced to an acceptable level or below through continuous hazard identification and risk management (ICAO, 2013). In other words, while risks always exist during operations, a given operation can be considered safe if the risk is managed to an acceptable level or lower. From this perspective, aviation safety refers to a state where various risks arising from the execution of flight operations or activities that directly support them are appropriately controlled, preventing accidents before they occur. This requires systematic and well-organized management efforts. If risks can be kept at or lower the level defined as acceptable, an operation can be considered safe. Consequently, aviation safety is the state in which risks associated with flight missions or supporting activities are effectively controlled to prevent accidents, requiring systematic and organized management efforts.
The issue of safety can be considered as an economic matter, as it involves the potential loss of human lives or properties. Thus, strong safety measures require practical management actions from an economic perspective. When risks are poorly managed and accidents occur, both human and material costs are incurred, including direct and indirect costs. Damage costs are calculated based on the damage applied to a person or a property. The ICAO recommends that safety issues should be seen as by-products of production activities or service delivery, requiring balanced and practical resource allocation to achieve both an organization’s production goals and safety objectives (ICAO, 2018).
Unmanaged safety leads to costs such as time loss, non-productive labor expenses, accident investigation and recovery costs, replacement staffing, reduced equipment efficiency, and potential fines or sanctions from authorities, all of which can severely impact an organization’s credibility.
In December 2013, the ICAO introduced Annex 19 on Safety Management, outlining aviation safety management standards and recommended practices for compliance by its 193 member states (ICAO, 2013). As a member state and council member of the ICAO, South Korea has explicitly stated its commitment to implementing these aviation safety management standards in Article 1 of the Aviation Safety Act, as outlined below (MOLIT, 2024).
“The purpose of this Act is to establish provisions concerning the methods for safe and efficient navigation of aircraft, light aircraft, or ultra-light flying devices, as well as the obligations of the state, aviation operators, and aviation personnel, in accordance with the standards and recommended practices adopted in the “Convention on International Civil Aviation” and its Annexes.”
In 1903, the Wright brothers achieved the first successful powered flight, and as the aviation industry developed, safety management became a longstanding practice. However, the ICAO introduced globally applicable safety management standards due to the increasing complexity of international aviation activities and systems, which diminished the effectiveness of traditional methods in maintaining aviation risks within acceptable levels (ICAO, 2018).
The ICAO described aviation safety management from the 1920s to the 1970s as a fragile system and focused its efforts on individual risk management and enhanced training, primarily based on accident investigations. Statistically, it was found that one accident occurred in every 100 flights. Also, safety management from the 1970s to the mid-1990s was described as a Safe system. During this period, safety management emphasized technology and regulation, relying on investigation results that included both accidents and incidents, with an average accident rate of approximately one per 10,000 flights. After the mid-1990s, the adoption of business management approaches to safety led to the widespread implementation of safety practices based on the routine collection and analysis of aircraft operational data, resulting in a reduction of the accident rate to approximately one per 1,000,000 flights. The aviation safety management methods introduced after the mid-1990s were ultimately consolidated into ICAO Annex 19, which has now become the aviation safety management guideline implemented by all aviation nations globally (ICAO, 2024).
Differences in both concept and methodology can be seen when comparing traditional safety management practices and the accident prevention measures introduced in Annex 19. Traditional safety management methods focused on investigating accidents or major incidents to identify their causes and prevent similar or identical accidents from occurring in the future. This approach to traditional safety management was primarily outcome-focused, as it emphasized whether aviation systems complied with design standards or not, based on the assumption that most aviation systems operated according to established design criteria. In contrast, the new safety management approach proposed by Annex 19 adopts the assumption that most aviation systems do not operate strictly according to aircraft design standards. It explains that the early identification and systematic management of practical drift, the difference between baseline performance and operational performance, is a key method for preventing accidents. Thus, the new safety management approach adopts a performance-based concept, emphasizing the outcomes produced during aviation mission processes.
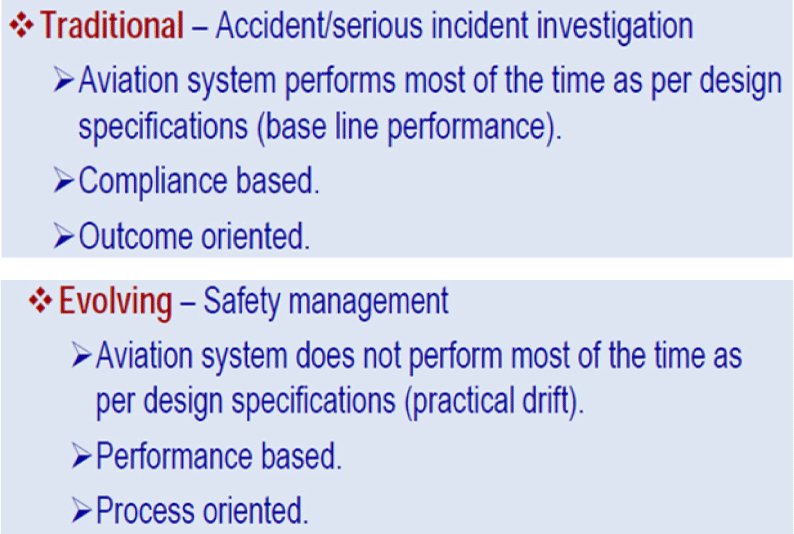
New safety management recognizes that aviation systems do not consistently operate as per design standards and emphasizes the need to acknowledge any discrepancies that occur during actual operations. Practical drift refers to the risks that occur during the execution of aviation missions. While traditional safety management aimed for zero risk, the new safety management approach acknowledges that risks are always present during mission executions but applies a concept focused on maintaining those risks within acceptable levels.
In the aviation sector’s Safety Management System (SMS), practical drift can be identified using three approaches: reactive methods, proactive methods, and predictive methods (Lee, 2019). The reactive approach makes corrections after an event has occurred and is primarily conducted through investigations. The reactive approach, while commonly used in traditional safety management, has the advantage of identifying not only the direct causes uncovered through investigations but also the contributing factors. The proactive approach focuses on identifying safety risks prior to system failures and implementing measures to mitigate them, using activities such as mandatory or voluntary safety reporting systems, safety inspections or audits, surveys, and other initiatives aimed at detecting hazards and minimizing associated risks. The predictive approach identifies hazards and assesses risks by analyzing various operational data that suggest potential safety threats. The predictive approach is exemplified by Flight Operation Quality Assurance (FOQA), which utilizes flight data, and Line Oriented Safety Audit (LOSA). Both proactive and predictive approaches are aligned in their objective of identifying risk factors and implementing mitigation measures prior to the occurrence of an accident. However, they are differentiated by whether the data used are obtained from safety-related activities or collected during routine mission operations. Additionally, identifying Practical Drift does not involve using just one method from the three approaches, instead, two or more methods may be used in combination.
III. Safety Management Measures for Defense Space Operations
Space force strength can be understood as the use of space assets, including reconnaissance satellites and satellite early warning systems, to maximize and refine the performance of advanced weapon systems and command and control systems on land and at sea. Therefore, safety management for space force strength should concentrate on activities related to the operation of advanced weapon systems linked to space assets such as military satellites, rather than adopting the broader concept of space safety, which includes the launch of space objects and the protection of crew members’ lives, as considered in the civil section.
South Korea’s national defense safety directive defines a safety accident as an event in which unintended causes result in harm to defense personnel or assets, or cause damage to public or civilian entities during the performance of defense missions or unit activities. They recommend that safety management activities should be carried out to protect defense assets from various safety accidents. Therefore, activities aimed at preventing damage caused by enemy forces intentionally attacking or destroying our space assets, such as military satellites, should be addressed strategically and tactically, as they fall outside the scope of safety management.
Through the review of previous studies, it was determined that the scope of safety management for the Republic of Korea’s space force strength includes preventing collisions between military satellites operating in space and space debris, as well as minimizing operational errors by space asset operators. Therefore, the safety management of space force strength should focus on the following two key elements.
First, efforts should be made to strengthen collaboration with the civilian sector and academia to prevent collisions between military satellites and space debris, and to develop specialized personnel within the military. The issue of space debris has become a major concern in the civilian space sector as well. Since the military has not yet independently developed satellites or launched artificial satellites into orbit, there are limitations in preventing collisions between military satellites and space debris. However, preventing damage caused by collisions between our space assets and orbital debris is within the scope of space force strength safety management. Therefore, it is essential to prioritize strengthening the scientific and technological collaboration with experts from the civilian sector and academia regarding satellite operations. The South Korean government is also participating in the establishment of international and regional space cooperation frameworks that address the issue of space debris. The Republic of Korea’s military should collaborate with the government and academia to support detailed aspects while simultaneously making efforts to ensure the safety of space force strength.
Secondly, preventing operator errors that may occur during the operation of space force strength is crucial. Based on the SMS concept required by ICAO, it is necessary to apply the four defensive mechanisms proposed by the Swiss Cheese Model in managing operator error. Through the lessons learned from major aviation accidents, the influence of military organizations, operators’ situation awareness, and the management of operational environments will be the key focus of the safety management. The operation of space force strength does not require a person to board and operate machine directly, rather it is carried out through remote response requiring correct judgment and action in various situations. Therefore, consideration of preventing human error will be a crucial focus of space force strength safety management.
Human space exploration began with the Soviet Union’s launch of Sputnik 1 in 1957, and in the early 1960s, when the United States announced its space projects, in which it became an area where the advanced scientific and technological capabilities of developed nations were applied. Since the beginning of the New Space era after 2000, both governments and the private sectors have participated in the commercialization of space, with more scientists and engineers contributing to the field, continuously developing knowledge and technology. However, the Republic of Korea’s military is now making efforts to enhance space force strength by utilizing space assets such as artificial satellites (Hwang & Choi, 2022).
The knowledge and technology required to prevent collisions between military satellites and debris in Earth’s orbit show that military capabilities are not on par with the civilian sector. By enhancing collaboration with the civilian sector and academia, the Republic of Korea’s military can address these gaps in capabilities.
The core concept of the Safety Management System (SMS), defined in ICAO Annex 19 acknowledges that the operational performance of aviation systems often differs from the baseline performance, with the goal of identifying and correcting the practical drift from the baseline performance as soon as possible. The measures required to accomplish this involve the use of reactive, proactive, and predictive methods. Each of these methods has its own distinct characteristics. However, what they have in common is that all involve identification activities based on safety information and data. The application of the SMS concept and operational deviation identification techniques from the aviation sector is also required in the context of space force strength operations. It is essential to establish a system that can continuously gather various data and information related to space force strength operations. In the aviation sector, a safety reporting system is implemented to ensure the availability of such data and information. It is important to remember that the reporter and the reported data/information are thoroughly protected in accordance with the law.
The Swiss Cheese Model is a safety management model designed to prevent safety accidents at the organizational level, particularly in large-scale organizations like the military. The safety management focus includes the four defenses that fall under latent conditions and active failures. The following are the management strategies for each defense mechanism that should be considered for the safety of space force strength:
Firstly, from the organizational influences perspective, resource management, including the crew selection, training, and assignment of personnel for space force strength operations, as well as the allocation of safety budgets and the acquisition of equipment and facilities, plays a direct role in ensuring safety. Additionally, it is essential to clarify the authority, responsibility, and the scope of delegation for operational personnel, as well as establishment of communication channels, clear operating procedures, regulations, and guidelines to improve collaboration within the organization.
Secondly, unsafe supervision should include measures to prevent inadequate supervision, such as the failure of supervisors to take necessary actions regarding operators. It is essential to manage the task organization and work schedules of operational personnel to ensure that the monitoring of space assets is not hindered by improper planning. Additionally, it is important to provide a means for freely reporting safety issues, and to ensure that reported risks are corrected in a timely manner. Furthermore, it is important to establish a voluntary reporting system for safety issues and ensure that reported risks are corrected immediately.
Thirdly, the preconditions for unsafe acts are essential to establish the capability to control physical and technological environmental factors, including operational environments related to space force strength operations. It is essential to prevent issues such as lack of sleep, fatigue, physiological impairment, and time pressure for operators, while making efforts to maintain their optimal physical and mental condition through proper management.
Fourthly, to control unsafe acts, also known as active failures, it is necessary to distinguish between errors and violations that humans may commit and establish appropriate management measures.
IV. Conclusion
Space assets hold significant military importance. However, if space assets cannot be utilized as military strength due to safety accidents, it would result in a significant loss of military capability. Maintaining space force strength during peacetime is essential to maximize national defense capabilities, which could result in the reduction of defense costs, a key objective of defense safety management.
The current concept of space force strength operation can be seen as commanding and controlling ground, maritime, aerial forces, and cyber warfare based on artificial satellites. As such, the safety management of space force strength focuses on preventing collisions between our satellites and space debris in orbit, as well as addressing operational errors caused by insufficient situation awareness and lack of knowledge among operators.
To protect the military’s space assets from space debris, collaboration with the civilian sector and academia should be strengthened. It is essential to establish and implement a medium and long-term plan to train military personnel capable of directly applying space knowledge and technology. Furthermore, to prevent operator errors, it is important to recognize that around 70% of aviation accidents are linked to human factors, and by drawing on lessons from aviation safety, potential solutions to address these issues can be explored.
Establishing space force strength requires physical and technological expansion efforts, such as securing various space assets and establishing organizational systems. However, one thing to remember is that safety issues are inevitably encountered during the execution of missions utilizing space force strength. This means that as the scope of missions utilizing space force expand, the number of safety issues will also increase. Therefore, safety issues must be carefully considered in order to strengthen space force strength.
This study adopted the Swiss Cheese Model that has been highly effective in the aviation sector, and in large organizations like the military. Failures in national defense space operations known as latent conditions such as organizational influences, unsafe supervision, and preconditions for unsafe acts remain dormant until they meet with active failures known as human errors. Therefore, to minimize active failures, it is crucial to identify and control latent conditions.
In future studies, it is expected that more diverse measures will be proposed if the national defense and space safety issues are carried out through other safety theories.